The Great Lactate Debate Part 2: can we ‘myth-bust’ the strong ion approach?
Jon-Emile S. Kenny MD [@heart_lung]
“The truth is rarely pure and never simple.”
-Oscar Wilde
In part 1, the crux of this ‘Great Lactate Debate’ was distilled into the unclear origin of the proton in the setting of ‘lactic acidosis.’ Is the [H+] secondary to biochemical work and ATP hydrolysis or is the proton from the addition of a strong anion [i.e. lactate] to the intracellular milieu? Note that this debate is not whether or not lactate can occur when mitochondrial respiration is normal [it can] or whether or not lactate is a bad actor [lactate is likely a beneficial adaptation for carbon shuttling between organs].
ATP Hydrolysis in Sepsis
In a brief missive submitted in response to the NEJM review on lactic acidosis, it was asserted that: 1. the strong anion lactate is the cause of the proton release 2. that ATP hydrolysis is less likely the etiology of proton elaboration because of a study on sedated sheep that employed whole-kidney MR spectroscopy to assess renal ATP turnover during experimental sepsis.
While the first point is discussed below, the second point strikes me as academically glib. First, the authors offer a sedated ovine model of sepsis assaying only whole-kidney ATP turnover. This is difficult to accept given that the kidney – and especially the renal cortex – acts more like the liver with respect to lactate metabolism. That is, the kidney tends to consume lactate. Studying an organ system like the lungs or muscle, which are thought to be lactate generators during sepsis, would have been more palatable. Secondly, a stable ATP value by spectroscopy does not necessarily implicate absent ATP hydrolysis, so long at ATP is being re-generated from ADP and/or phosphocreatine. Indeed, and somewhat ironically, the very same authors of the aforementioned letter to the NEJM penned an excellent review of hyperlactatemia in sepsis in Critical Care Medicine. In this review, the authors pay special attention to robust in vitro and human in vivo data demonstrating that the Na+/K+ ATPase is a key pathophysiological driver of sepsis-associated hyperlactatemia. It should not escape the reader’s attention that the ATPase enzyme, by definition, hydrolyzes ATP [and therefore elaborates protons – see below]. Further, the equation for glycolysis given in part 1 reveals that ADP is a substrate for pyruvate [and therefore lactate] production. Without ADP, glycolysis cannot proceed, just as glycolysis could not proceed with deficiency of any other substrate. In order for there to be ADP as a substrate – for glycolysis and therefore lactate production – ATP must be hydrolyzed to ADP. Furthermore, in addition to the Na+/K+ ATPase, the activation of adenylate cyclase by epinephrine ultimately leads to the hydrolysis of 2 phosphate bonds [and therefore 2 protons] as ATP becomes AMP [see figure 1].
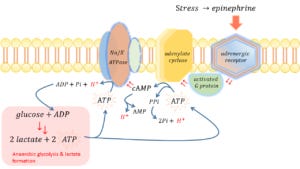
Figure 1: Schematic of adrenergic-induced biochemical work at the cell membrane; hydrolysis of ATP by ATPase & adenylate cyclase elaborate protons [H+ in red]. ADP is a substrate for glycolysis and the ATP generated by glycolysis may then be used to drive the biochemical work at the membrane. ATP is adenosine triphosphate, ADP is adenosine diphosphate, AMP is adenosine monophosphate and cAMP is cyclic AMP. Pi is inorganic phosphate and PPi is pyrophosphate. cAMP stimulates the Na/K ATPase; not pictured is that cAMP also stimulates glycolysis [highlighted in red bottom left]; cAMP acts as a second messenger to stimulate many cellular processes. Hydrolysis of cAMP to AMP is via the enzyme phosphodiesterase – of which there are many inhibitors, including caffeine. PPi is hydrolyzed by the – not pictured – enzyme pyrophosphatase.
In a particularly elegant study [also reviewed here], septic patients had micro-dialysis probes placed in their quadriceps muscle beds. The authors found a muscle-to-arterial lactate gradient that was abolished when Ouabain [a Na+/K+ ATPase-specific inhibitor] was titrated, locally, through the microdialysis catheter. A similar, in vitro, study also revealed that Ouabain abolished the fall in intra-cellular pH. As highlighted in figure 1, activation of the Na+/K+ ATPase is mediated by B2 stimulation which explains why lactate rises in response to both adrenergic stress and catecholaminergic agents [e.g. albuterol]; as well, this clarifies why lactate falls when adrenergic tone is attenuated, for example in response to intra-venous fluids or beta-blockers.
In totality, it is hard to believe that ATP hydrolysis is not occurring, despite MR spectroscopy revealing constant levels of ATP in septic sheep kidney. Despite these plausible mechanisms for ATP hydrolysis and proton elaboration in sepsis, it does not mean that the Stewart approach is an invalid explanation for the contemporaneous decline in pH observed during high glycolytic flux and rising lactate.
Recovering Stewartite
I first learned about the Strong Ion Difference [SID] on rounds in the ICU about 10 years ago. I was so intrigued by this paradigm that I marched down to Bobst Library and checked out Stewart’s original textbook [I had been the first to do so in at least a decade]. I read it cover-to-cover and I was completely sold; then I started to proselytize my co-residents. The Stewart approach made sense, and I liked the way it explained hyperchloremia-associated metabolic acidosis. Further, I wanted this theory to be true because:
it was disruptive
Peter Stewart was Canadian
I figured that primordial cells probably started to regulate strong ions in the salty oceanic soup before protons, so there was an evolutionary plausibility to it
At this time, Dr. David Goldfarb passed to me a newly-published critique of the Stewart approach, which I also read voraciously.
This paper, by Kurtz et al. is an imperative read for those who find the Stewart approach to be the ‘obvious truth.’ It is well-written and ceaselessly scientific. It details many of the mechanistic problems with the Stewart approach – specifically how different strong cations can alter the structure of water in completely opposing ways [e.g. Na+ is a kosmotrope and K+ is a chaotrope], how ions have been shown to have minimal effect on the hydrogen-bond network of water, as well as how proteins can alter [H+] without any obvious change in the 3 independent Stewart variables [e.g. bacterial rhodopsin creates proton gradients in response to photons of light]. Further, Kurtz et al. make clear that one should not simply accept macroscopic, mathematical electroneutrality as an ‘obvious fundamental of physical chemistry;’ this assertion is an epistemology – not ontology – and the two should not be confused. Macroscopic electroneutrality – as defined by the SID – is not obvious, and may be locally disobeyed within the cell. Microscopic charge gradients certainly occur and are described by the Debye Length – a function of ionic strength, not the SID! Additionally, the physical chemistry described by Debye does play a role in thermophoresis – an intriguing phenomenon – described next.
In a fascinating paper published in Nature Communications about 2 months ago, the Stewart paradigm for explaining pH is left wanting. In their brief communication, Keil et al. describe how primordial proton gradients may have been generated simply by the application of thermal energy. In their study, a phosphate buffer solution had an external, thermal gradient applied. Accordingly, this solution had – macroscopically – stable [Atot], SID and PaCO2; yet over a period of hours, proton gradients were generated with pH differences up to 2.0 [see Figure 2]!
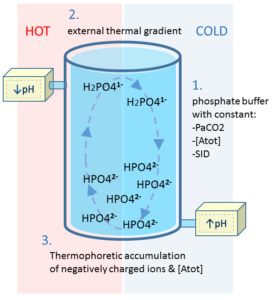
Figure 2: Cartoon of experimental results of Keil et al. Thermophoresis of an ionic species is described by the Soret Coefficient, itself, mediated by the Debye Length of the charged species. This defines the distance over which electroneutrality may be violated. There is an accumulation of negative charge & [Atot] at the base of the column, but this was where pH was the greatest; i.e. most alkaline - counter to what the Stewart approach predicts.
As illustrated, the external thermal gradient caused separation of ionic species by convection and thermophoresis [i.e. the movement of molecules in a temperature gradient]; this favoured the accumulation of both hydrogen phosphate [HPO42-] and dihydrogen phosphate [H2PO41-] at the bottom of the column, but the former much more than the latter. The devout Stewartite might argue that while macroscopic electroneutrality [i.e. the SID] was stable in the solution, microscopic gradients were formed. Paradoxically, despite more negatively charged species accumulating at the bottom of the column [-2 charged hydrogen phosphate, recall from part 1 that Pi has strong anion characteristics], the bottom was where the pH was most alkaline. Additionally, if we consider both HPO42- and H2PO41- species as [Atot] in the Stewart paradigm, their accumulation at the bottom of the column should, like a diminishing SID, result in a local acidosis. Nonetheless, the rise in [H+] was actually at the top of the column – away from where the SID was shrinking and where the [Atot] was rising.
While the findings of Keil et al. seem to fly in the face of the Stewart paradigm they may be explained if one simply counts protons. [H+] fell at the bottom of the column because the following equation was pushed leftwards by the thermophoretic accumulation of hydrogen phosphate [HPO42].

Conclusion
The Stewart approach is mathematically pleasing and describes change in pH just as well as Henderson-Hasselbalch, yet when it comes to the actual physical chemistry of acid-base – the paradigm from which it is derived and should champion – it bears inconsistencies, especially in light of the critiques by Kurtz and colleagues and the thermophoretic proton gradients recently described by Keil et al. The SID relies on the ‘obvious’ need for electroneutrality; yet this is neither obvious, nor universal. Additionally, it’s possible that evolution needn’t ‘regulate’ strong ions first – it could have been protons near a thermal vent! For these reasons, I consider myself a ‘recovering Stewartite.’ If I return to Carl Sagan – as I often do – I’ve yet to encounter ‘extraordinary evidence’ that the Stewart approach to pH is universally valid. In fact, as a non-physical chemist peering through the open space of the Debye Length, I see the contrary.
Best,
Dr. Kenny is the cofounder and Chief Medical Officer of Flosonics Medical; he is also the creator and author of a free hemodynamic curriculum at heart-lung.org